
Figure 1 - Correct technique of the volleyball spike
What are the biomechanics of a volleyball spike and how can we optimise it's power and accuracy?
A volleyball spike is one of the most important and powerful attack moves in the game of volleyball. (shown in figure 1) The aim of the 'spike' is to hit a ball over the net into the opposition's area, creating enough power in a downward motion for this move to win the point.The power that is generated into the ball can be maximised through the analysis of biomechanical principles to efficiently execute a spike with adequate speed. Thus this blog will demonstrate the biomechanics behind a volleyball spike and why we need force, gravity, acceleration, power and accuracy to produce an optimum spike in volleyball.
Biomechanics' is the science involved with the study of forces acting on and within a biological structure and the effects produced by such forces; the primary purpose of biomechanics is to evaluate the laws and principals of mechanics about human performance in order to gain greater in-depth understanding and knowledge about specific details (Blazevich, 2010).
The four key components consist of preparation/take-off, jumping height, arm swing and the hit/follow through. In order to generate the greatest amount of power when spiking, a volleyball player needs to be able to combine these forces into one creating a flowing movement. The biomechanics of these components will now be explained in more detail.
Preparation/Take off - What are the biomechanical principles of the 'take off' phase?
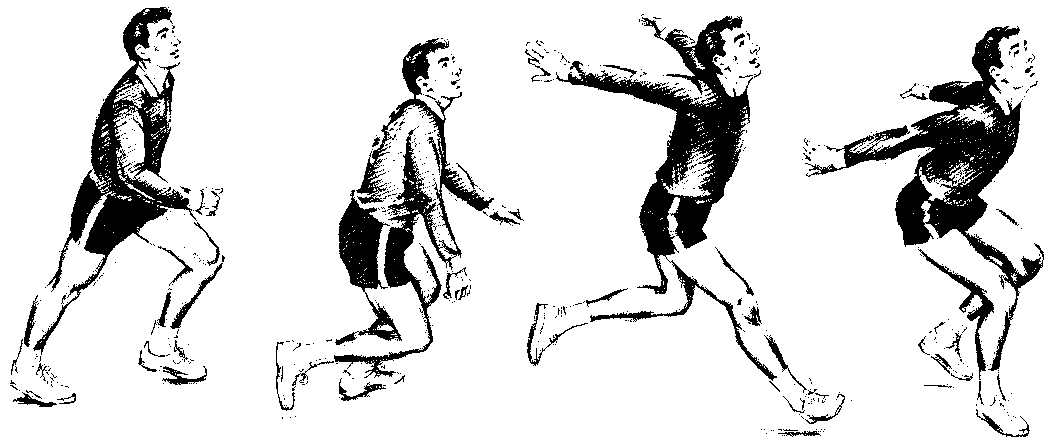
Figure 2 - Ground reaction forces on foot in relation to the arm movement
The pre-execution phase of the volleyball spike is the running phase and the take-off. As shown in figure 2, the running approach is important to give the volleyball player maximum momentum for the height of the vertical jump (Moellendorf, 1999). It is recommended to plant and take off quickly during an approach. This is due to the concepts of kinetic energy and potential energy. During the approach the body has kinetic energy, described as energy in motion, faster moving objects will have greater kinetic energy (Blazevich, 2010). The goal is to transfer this kinetic energy into potential energy in preparation for the jump. If it comes to a stop the kinetic energy will be less, therefore, preventing jumping as high. Since potential energy is the product of the mass of the player, gravity, and the height of the jump, the height is what determines how much potential energy can be attained (Blazevich, 2010).
Jump - What are the biomechanical principals of the 'jump' phase?
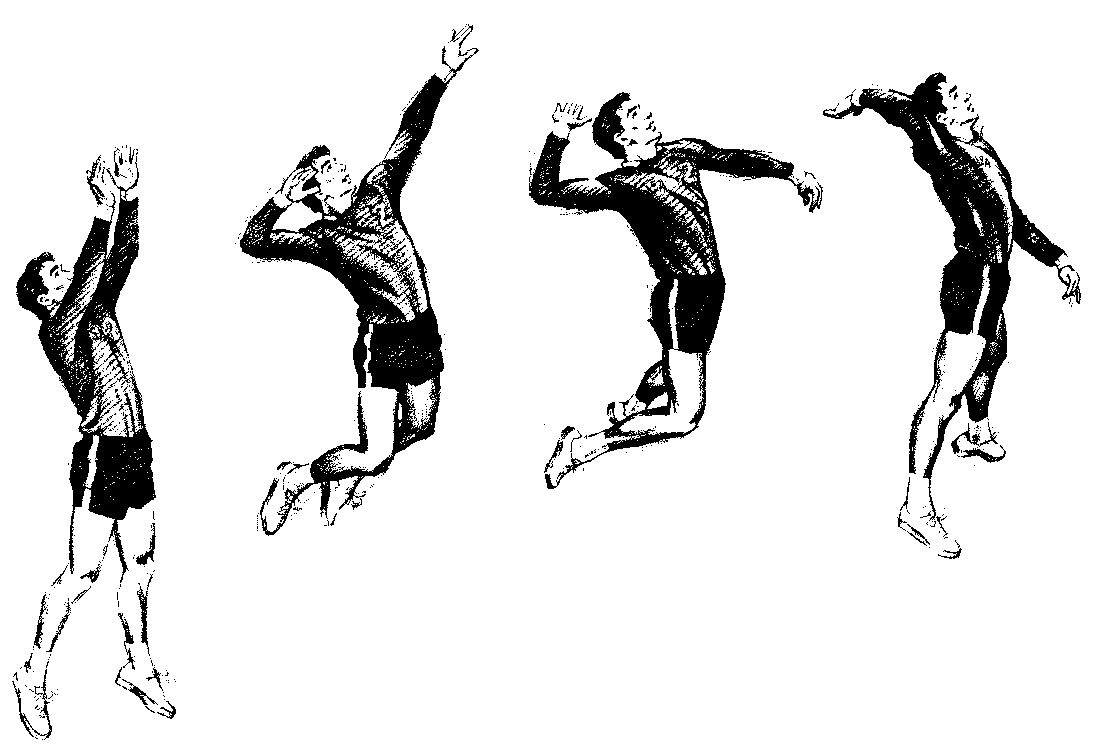
Figure 3 - Projectile into the jump in relation to arm positioning
This is where Newton’s Third Law of Motion comes into play, ‘For every action there is an equal and opposite reaction’. The force built up from the take-off is applied to the ground with both vertical and horizontal components. (as shown in figure 3) This force is then exerted back into the athlete, propelling them forward and upward (as shown in figure 4), if the force is large enough to overcome our inertia.

Figure 4 - A vertical force applied when the foot contact's the ground.
The relationship between the kinetic (force) energy and Newton’s Third Law is what creates a greater jumping height. Kinetic energy is the energy associated with velocity of our body. The power of the jump comes from the vertical force generated from the foot plant and push-off of the legs, while the transfer of momentum is due to the direction of the foot plant and the use of the arm swing (Blazevich, 2010). To measure the kinetic energy of an athlete will improve the efficiency of the jump, an athlete kinetic energy in relation to their heart rate reserve is worked out with the following equation:
HRR = (HRcurrent – HRresting)
÷ (HRmax – HRresting) × 100
= (140 – 60) ÷ (180 – 60) × 100
= 67.7%
KE = 1
⁄2mv2 = 1
⁄2 × 75 × 2.42
= 181.5 kgm2
KE:%HRR = 181.5/67.7 = 2.68/. (Blazevich, 2010)
This is important for maximum energy and power for the next phase. This process can also be referred to as a summation of forces.
Arm Swing - What are the biomechanical principles of the 'arm swing' phase?
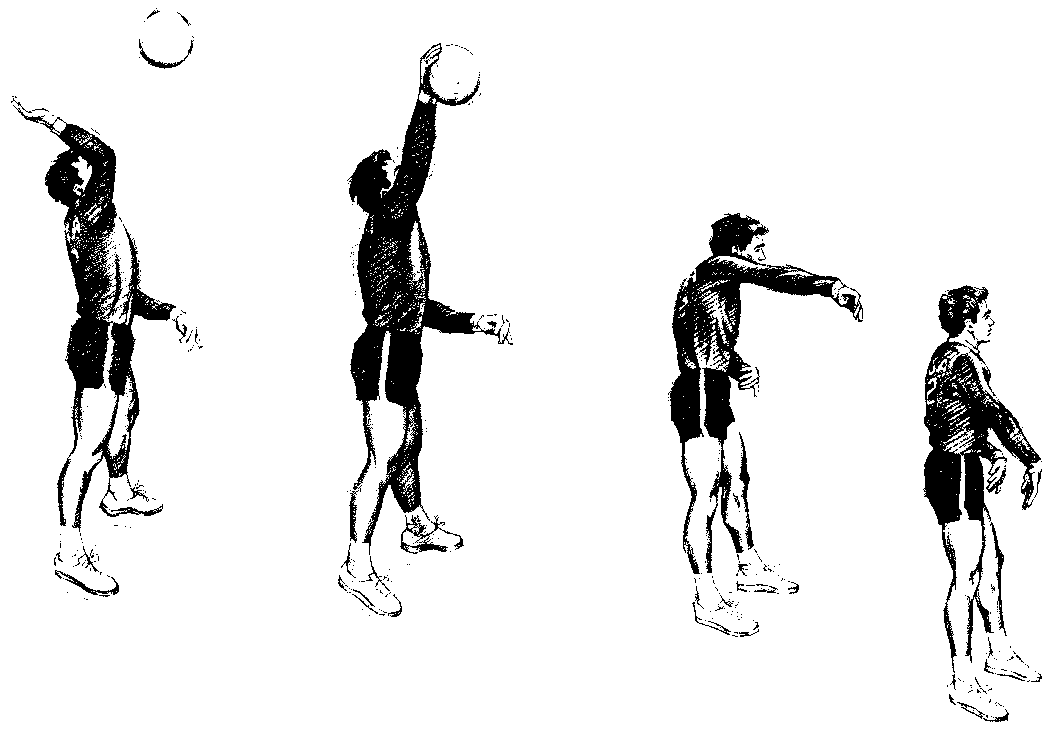
Figure 5 - Arm swing in relation to the follow through
The motion the arm's swing contributes during the jumping phase. It is one of the most important factors that develops the height of the jump. "That is,
the increased range of motion for the arm
swing in addition to the earlier time of
initiation allowed the arm to generate more
energy, which is transferred to various body
points to improve vertical jump performance (C. Hsieh & G. Heise, 1997)". (as shown in figure 5) The arm is initially left behind the body in a hyper-extended position, this is to prepare for the best forward position when the arms are swung forward, upward and then downward. When the arms are in the upward acceleration phase, they push against the inferior parts of the body to increase the downward force with acts upon the floor (creating a greater counter reaction on the athlete). Generating higher amounts of vertical momentum will dictate the height of the athlete’s centre of mass at take-off. The arm momentum and position, along with truck rotation and flexion also play a part in the ball velocity (Moellendorf, 1999).
Hit/Follow Through - What are the biomechanical principles of the 'hit' phase?
The aim when spiking the volleyball is to transfer the force that has been produced by the above phases into the ball to create maximum speed. When making the connection with the volleyball, the moment of force (torque) is important to take into consideration. This relates to the force acting on the ball which causes it to rotate. This is measured by the equation ‘torque = inertia x angular acceleration (t=la)’ and will play a huge part in ball placement. (Blazevich, 2010). Another important biomechanical principle which relates to the ball placement is The Magnus Effect, which refers to the relationship between air pressure and the ball (Blazevich, 2010). shown in the figure 6 below
.

Figure 6 - Magnus Effect on a volleyball.
Figure 7 - The movement phase of the volleyball spike
The Answer
To effectively generate the greatest amount of power when spiking, a volleyball player needs to perform these key components fluently and combining the forces as one. In the take off phase, the kinetic chain allows for this sequential acceleration of the trunk, torso and limbs during the spiking action, balancing the kinetic energy is vitally important to ensure efficiency. In the jump phase, the power can be optimised in a volleyball spike by jumping higher, in turn, applying a greater force against the ground will increase the height of the jump. In the hit phase, the contact point of the ball and hand was found to provide the power and accuracy at the peak of the jump, producing the most speed with contact higher on the ball, with angular momentum used by the athlete to transfer the most power on to the ball. The Magnus effect was also another factor in producing power and accuracy and for players to know how this factor affects the ball in play. It is evident that the understanding of the biomechanical principles can lead to the efficient and effective spiking technique being delivered. It is the role as teacher/coach to understand these biomechanical principles to express and apply these principles to an athlete’s chosen sport to maximise the effectiveness and efficiency of the chosen skill sequence while minimising the chance of injury occurring.How else can we use this information?
This information can be used to improve the technical elements from a biomechanical approach to the volleyball spike. Using the biomechanical approach to sport skills allows for coaches/teachers/athletes to quantitatively and qualitatively develop skill teaching cue's and purposes behind certain techniques.
An understanding of these biomechanical principles allows for further in-depth knowledge of how to improve results within a volleyball game context, which can in turn, also be applied to other sports involving jumping such as for high jump, arm swinging motions such as for fast bowling in cricket and projection of a ball such as pitching in baseball just to name a few.
References
Blazevich, A. (2010). Sports biomechanics the basics: Optimising human performance (2nd ed.). A&C Black Publishers.
Hsieh, C., Heise, G. (1997). Arm swing of the volleyball spike jump performance between advanced and recreational female players. Health Uottwa. University of Texas, Pan American, Edinburg, TX, USA. Retrieved 18th June 2015 from http://www.health.uottawa.ca/biomech/csb/Conference%20Proceedings/NACOB/Abstracts/306.pdf
Moellendorf, S. (1999). The Physics of Volleyball. Physics Volleyball. Retrieved 18th June 2015 from http://www2.hesston.edu/Physics/Volleyball/paper.htm
Hsieh, C., Heise, G. (1997). Arm swing of the volleyball spike jump performance between advanced and recreational female players. Health Uottwa. University of Texas, Pan American, Edinburg, TX, USA. Retrieved 18th June 2015 from http://www.health.uottawa.ca/biomech/csb/Conference%20Proceedings/NACOB/Abstracts/306.pdf
Moellendorf, S. (1999). The Physics of Volleyball. Physics Volleyball. Retrieved 18th June 2015 from http://www2.hesston.edu/Physics/Volleyball/paper.htm
No comments:
Post a Comment